by Tom Bales and Euclid Laboratories
Tom Bales is an American entrepreneur and inventor. He is the co-founder of two medical device companies, Symbiosis Corp. and Syntheon LLC, and an aerospace company, EAC. Bales has robotics teams and taught STEM subjects. He has worked with teams of students to build a global array of cosmic-ray detectors, the “world’s largest telescope” known as ERGO (Energetic Ray Global Observatory). Bales and his family have been competitors on the “Battlebots” television series (ABC/Discovery) for the last four seasons. And following his interest in horology, Bales founded Derek Pratt watches.
Bales recently sent Ian Skellern some detailed information about a Time Traveler’s Clock he has made. Below is an edited, abridged version of the text.
Time Traveler’s Clock (TTC)
by Tom Bales and Euclid Laboratories, Inc.
“A man with a watch knows what time it is. A man with two watches is never sure.” – Lee Segall
“A man with three clocks is surer than a man with two clocks.” – Tim Van Baak
Origin of the TTC, how did this project begin?
Imagine that after discovering and rebuilding the (imaginary) Time Viewer originally constructed by Leonardo da Vinci, an inventor saw the need for a navigational clock for time travelers. Imagine that Leonardo traveled to America in the 1940s and wanted to return to Milano in 1478, so he built a Time Viewer to learn the future technology needed to construct a time-traveling machine.
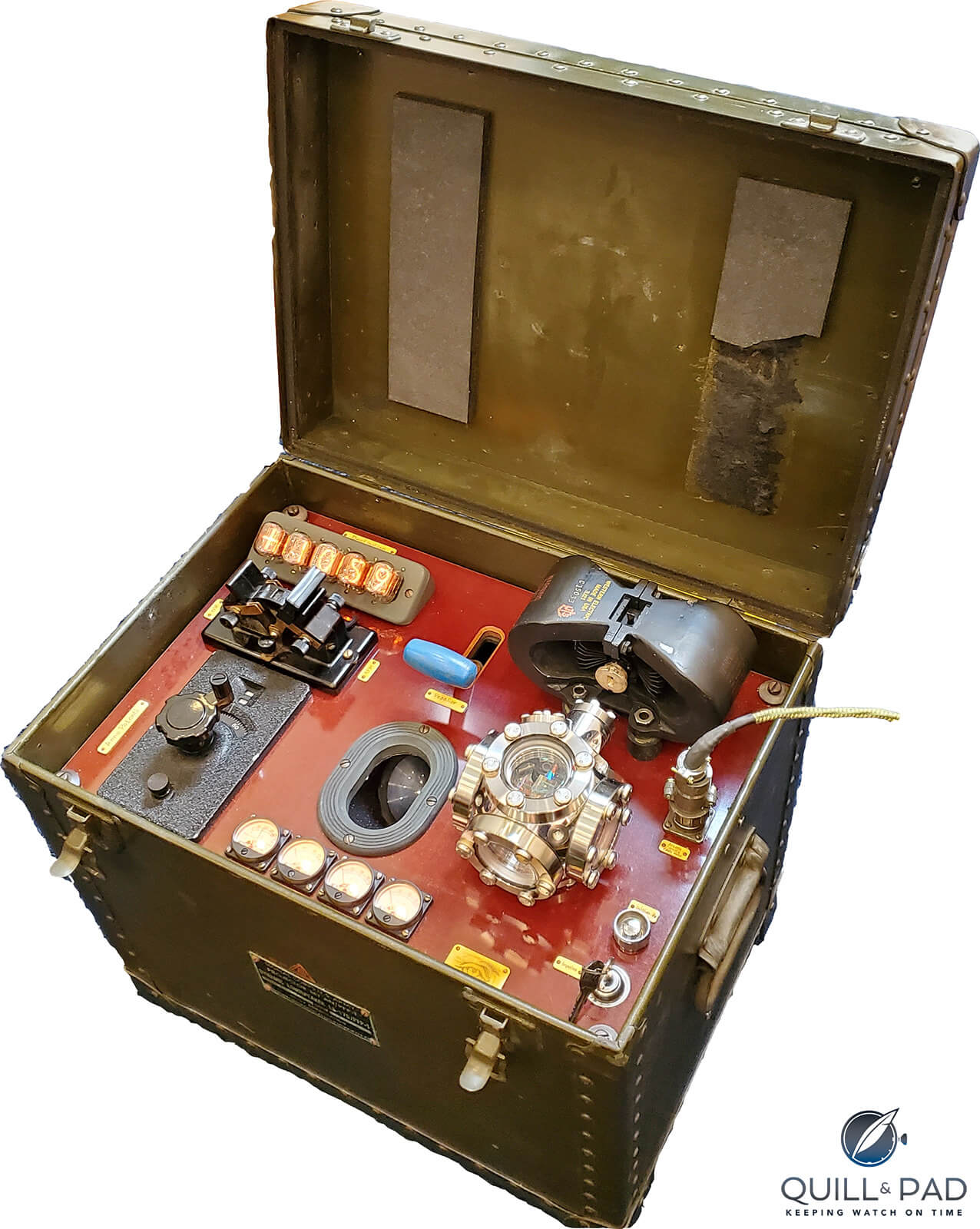
Leonardo da Vinci’s imagined Time Viewer
We know that Leonardo lived in Italy for the rest of his life. Could it be that he couldn’t navigate in time anymore since he lacked a chronometer? If an inventor is ever able to build a time-traveling machine, he would want to carry with him the most reliable possible chronometer to keep track of where he is and where he is going.
I decided to create that timekeeper.
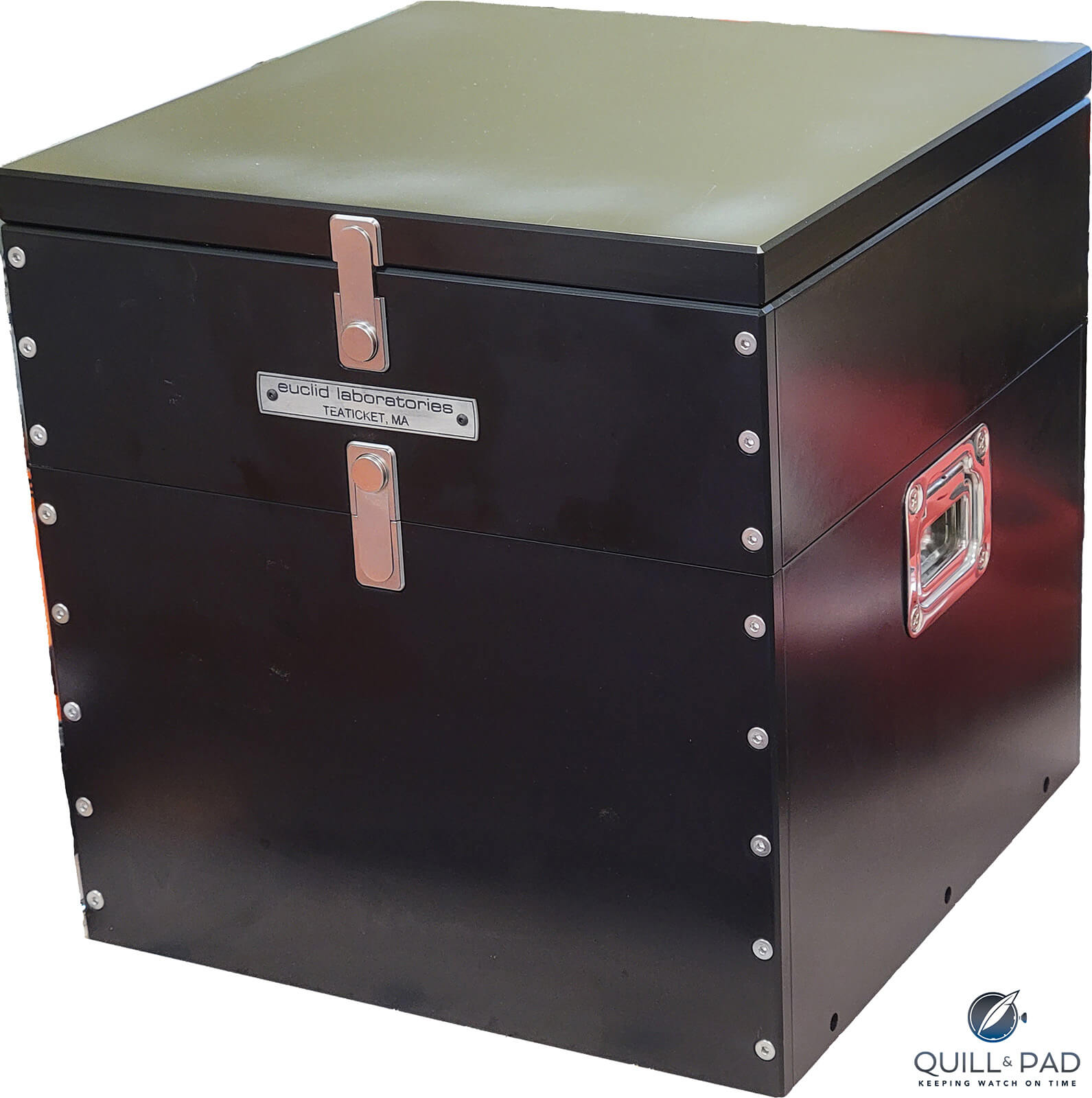
Time Traveler’s Clock case closed and ready to travel
Why do you need a Time Traveler’s Clock?
Imagine you’re traveling in time and space. Just like navigators of old, you’ll want to have some “bearings” back home so that you can return or go to a new destination. Time is essential to navigation and we can assume that UTC (Coordinated Universal Time) will always be a good time to know. But, you never know – you might end up in the past when there was no GPS. If so, you would want an atomic CSAC (Chip Scale Atomic Clock) to keep you navigating. Or you might end up before there was electricity, so a manual-winding marine chronometer would be handy as well
You might travel to a time near the present with electricity but the GPS system isn’t operational. You might travel to the future when the time standards and navigational systems are completely different from what we have now. However, you should still be able to find some form of electricity to keep your batteries charged to maintain an internal GPS clock and an atomic-stabilized chronometer.
The sands of time may eventually cause the GPS and CSAC systems to fail, so you’d want a silicon RTC clock as well. And if all of the electronics fail, a reliable Hamilton marine chronometer will still work for many years.
What if time travel is impossible?
Perhaps time travel, either to the past or the far future, won’t turn out to be possible. If so, a discerning traveler would still want to be able to navigate by land, sea, or air via GPS or using a sextant and atomic clock. Or a backup silicon chip clock, or even just a mechanical chronometer.
The TTC has you covered for any imaginable eventuality. And if you’ve spent a lot of money on your yacht or aircraft, you’d want to have the absolute, ultimate, most reliable timekeeper available.
Before the Time Traveler’s Clock there were prototypes (many prototypes)
Cesium Breadboard Prototype
The first experimental design was constructed with a Hamilton 21 chronometer, a Microchip/Jackson Labs cesium chip-scale atomic clock (CSAC) (later used in the MCB Prototype), a uBlox GPS evaluation kit (EVK-M8T), a Maxim DS3231 real-time clock (RTC) chip, and a servo mechanism to rotate the chronometer by a small amount (about one half degree of arc) following the one-second pulses from the GPS and CSAC.
This prototype was used to determine if it was possible to resonate a mechanical chronometer, how much motion would be required, and to refine the displays for time from the GPS and the RTC. A circuit was incorporated to allow resetting the RTC from the GPS whenever desired.
Winding mechanism prototype
The mechanism that continually winds the chronometer required considerable development. Automatically winding the chronometer requires substantial torque. The state of wind must be periodically measured to determine if winding is required. As the chronometer runs down, the winding input stem must be allowed to rotate backwards so the chronometer may continue operation. Finally, provision must be made for manually winding the chronometer.
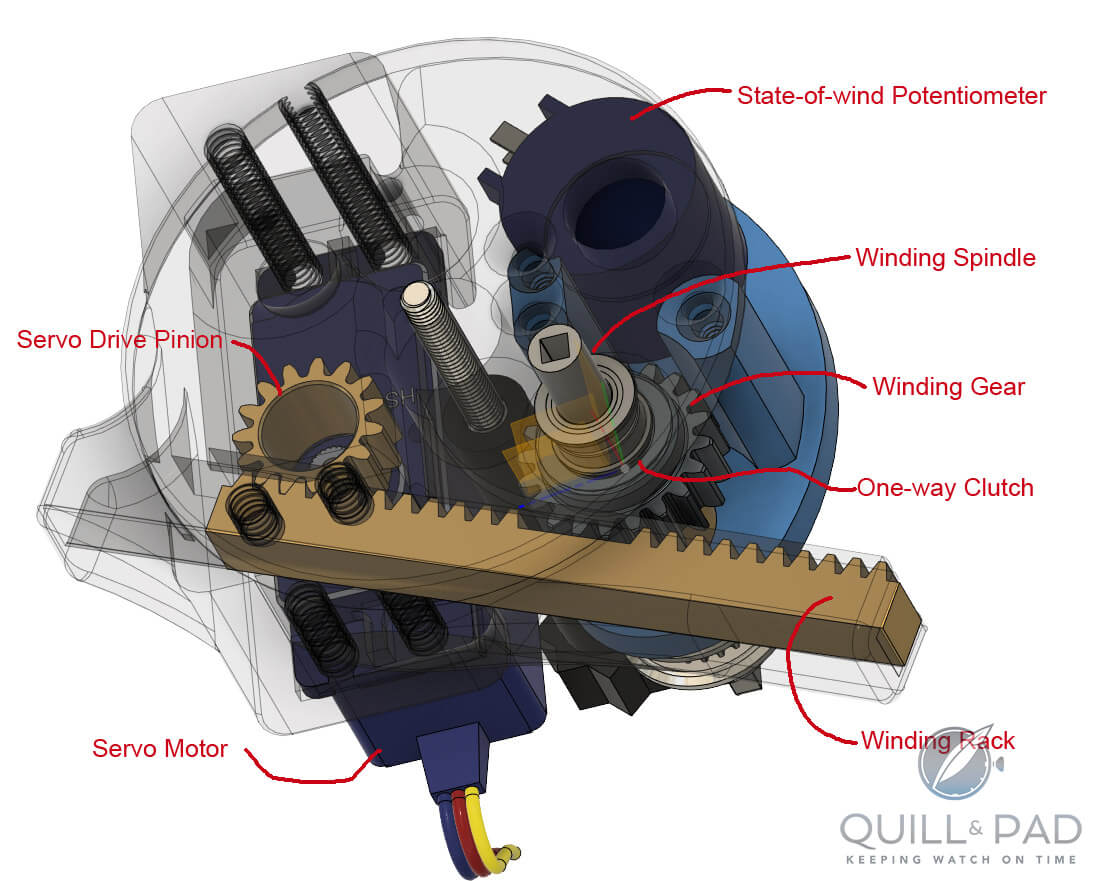
Time Traveler’s Clock marine chronometer winding mechanism
The successful winding mechanism uses a titanium-geared, radio-control servo motor fitted with a drive pinion. The drive pinion is driven alternately clockwise and counterclockwise by the servo motor. The servo drive pinion is constantly meshed with the winding rack, which is driven back and forth by the servo motor. The winding rack engages a winding gear. The winding gear rides on the winding spindle, which has a square opening on its upper end to engage the winding stem of the chronometer.
Every hour, the condition of the state-of-wind potentiometer is measured by the microcontroller and the servo motor is actuated back and forth until the chronometer is fully wound.
Pyramid Cesium CSACGPSDO prototype
The next generation of prototypes was built in a pyramidal shape with proportions from the Great Pyramid of Giza. The bottom of the enclosure was black acrylic with a window for the electronic displays, and the upper cover was clear acrylic to allow viewing of the controls and chronometer. The thermal printer to record the natural rate of the chronometer was added to the rear panel, and backup batteries (Saft thionyl-chloride D cells) were fitted inside the base.
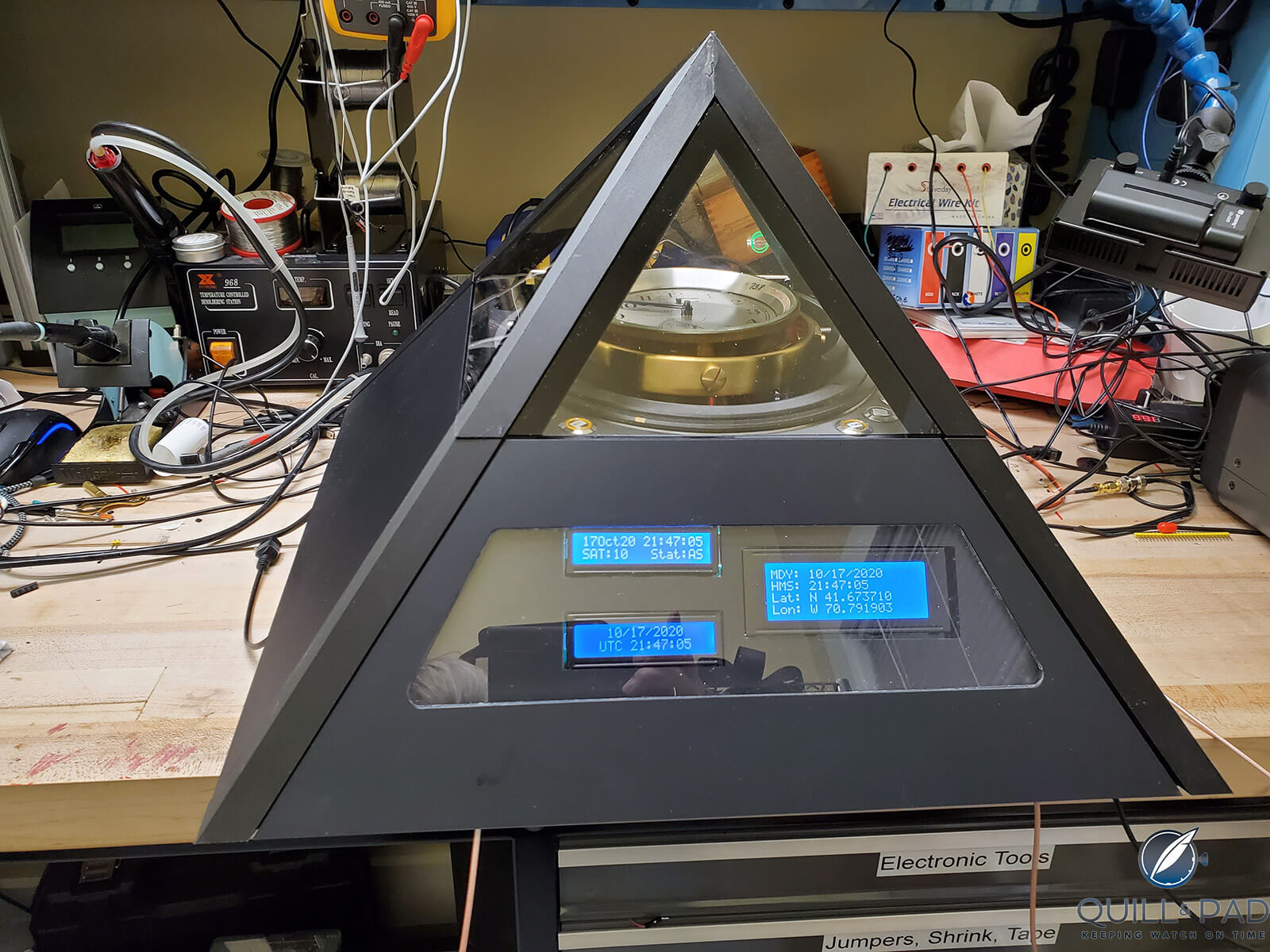
Pyramid cesium prototype
In the Pyramid Clock, the atomic reference was a Jackson Labs cesium-CSAC GPS disciplined oscillator module, which incorporated a cesium reference oscillator phase-locked to the GPS chip. A separate display was run from this module to display the date, time, latitude, longitude, and operational characteristics of its GPS and the CSAC.
Cube Box rubidium prototype
I decided that the pyramid enclosure, while having an interesting appearance, was not very space efficient and decided to go with a more traditional, cubical, mahogany box, similar to those traditionally used for marine chronometers, but increased in size.
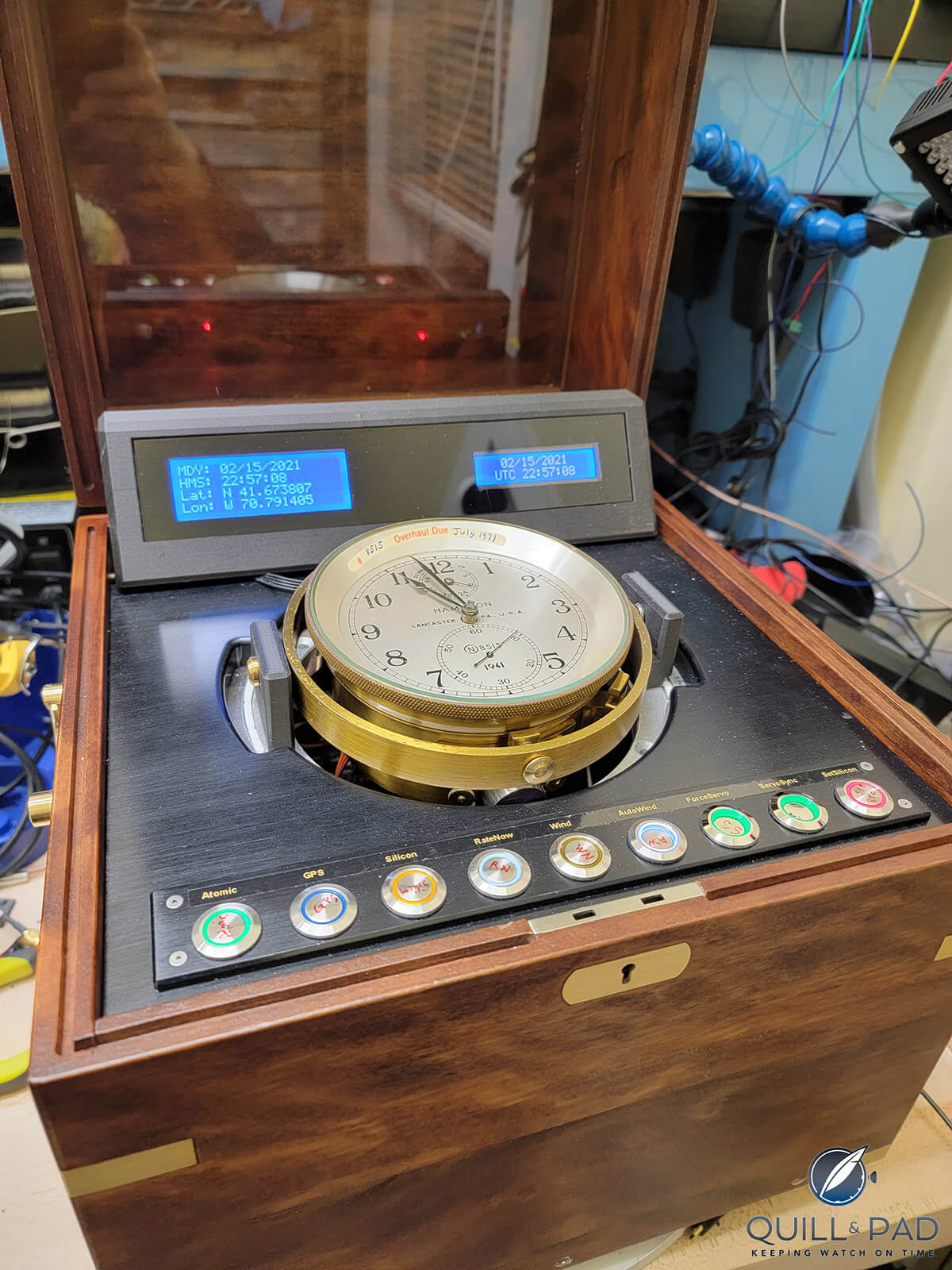
Time Traveler’s Clock wooden box prototype
In this prototype, the atomic reference was a standalone rubidium miniature atomic clock (MAC) from Jackson Labs. This oscillator was calibrated with a GPS-disciplined reference clock before use.
This simpler design works as well as the cesium CSAC/GPS disciplined oscillator module, is less expensive, and is less complicated.
Refinements needed to this design included improving the reliability of the automatic winding system, providing a more precise gimbal support bearing, aesthetics, and reversion to a cesium-based atomic reference.
Metal Cube Box final prototype
The Metal Cube Box prototype incorporated a fully machined anodized aluminum enclosure in place of the mahogany box following the same design and dimensions.
The displays were simplified to two LCD displays: one showing the GPS date, time, latitude, and longitude and one showing date and time from the RTC. No separate display was used for the CSAC, as it operates only to supply the 10 MHz precision reference frequency.
The rubidium MAC was replaced with the cesium CSAC used in the Cesium Breadboard Prototype. Otherwise, the MCB Prototype was like the Cube Box Prototype and used the same electronic hardware and firmware. The operational scheme remained with the GPS module being fed a precision 10 MHz frequency reference from the CSAC to ensure long-term accuracy during holdover.
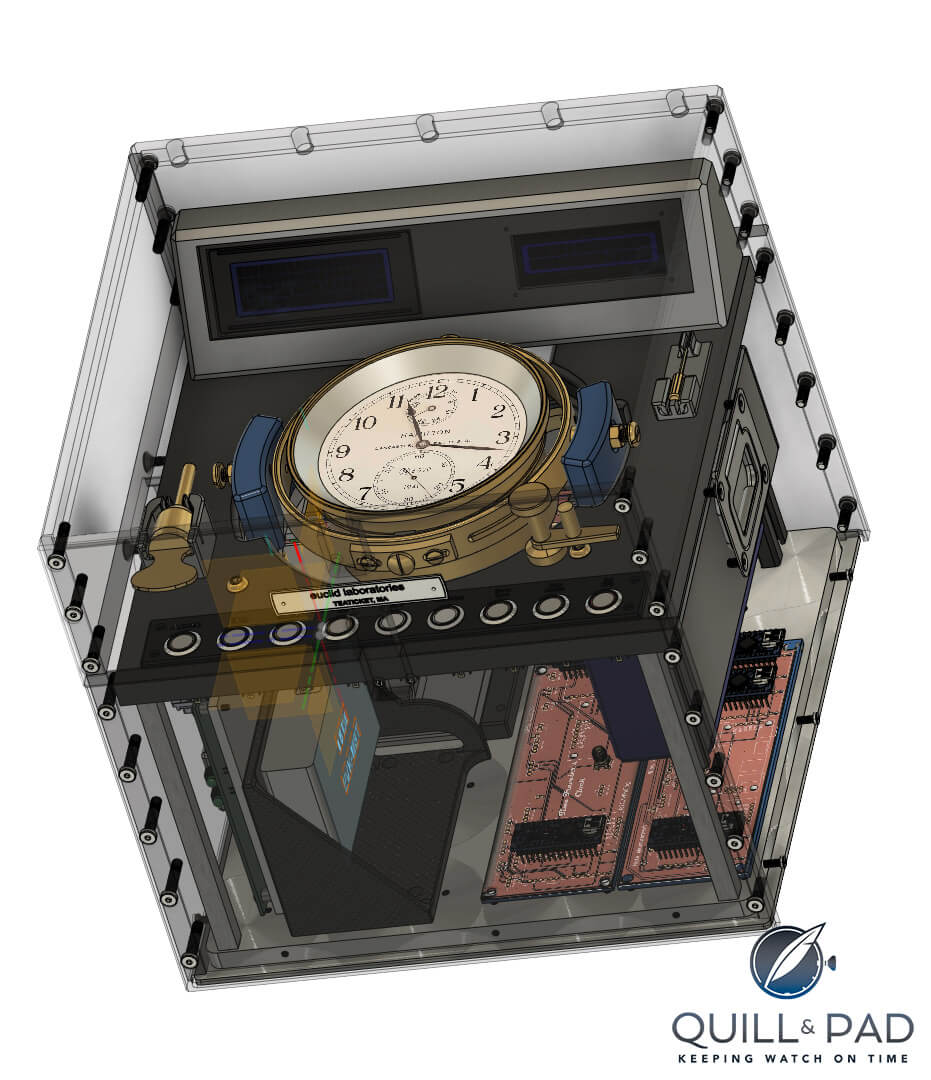
Rendering of the Time Traveler’s Clock
How the Time Traveler’s Clock works
Marine chronometer advantages and deficiencies
The Hamilton 21 Marine Chronometer represents the height of development of portable mechanical clocks for navigation. The basic design, developed by several companies in Switzerland (Zenith, Ulysse Nardin, and Ditisheim among others) includes a wooden box, gimbals, chronometer case, and a movement that incorporates a fusée mechanism for near-constant winding force as the mainspring winds down, a detent escapement, and a temperature-compensated balance wheel fitted with a helical hairspring.
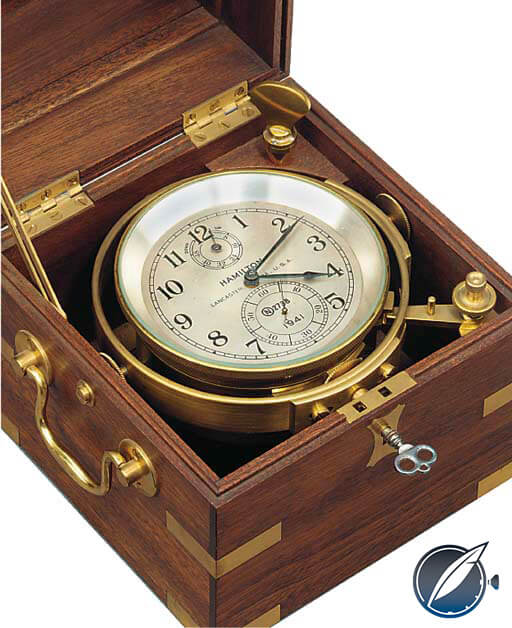
Hamilton 21 marine chronometer
When the U.S. Navy realized the upcoming need for thousands of such chronometers in the years prior to World War II, the only company that could fill the need was Hamilton Watch Company of Lancaster, Pennsylvania.
Hamilton could meet the need if the basic design of a Ulysse Nardin chronometer was exactly copied except for the balance wheel assembly. Hamilton had developed a better way to create a temperature-compensated balance wheel that would not require the time-consuming and laborious process of regulation that was required by the Swiss chronometers.
The advantages of a mechanical, manual-wind chronometer are its reliability and trustworthiness. As long as the chronometer is wound each day, accurate time is assured. Each instrument could be regulated to keep time within a second per day, and – more importantly – its deviation from perfect time would be a very precise value that would not change over the life of the chronometer.
Each chronometer supplied to the Navy (and also to the U.S. Army Corps of Engineers) came with a record showing when it was set to time as well as its measured error per day. From these data a navigator could subtract the daily error multiplied by the number of days from the currently displayed time, resulting in a very accurate “corrected” time for navigation. Weaknesses of a mechanical chronometer include the need for continual winding; and, if the instrument is allowed to run down, then all knowledge of time will be lost. Also, while physically very robust, these instruments have to be handled with great care in order not to upset the timekeeping. On larger ships the navigator kept two or three chronometers for redundancy.
During normal operation, the Time Traveler’s Clock keeps the mechanical chronometer synchronized to GNSS (GPS) atomic precision by means of a servo-mechanical system. It was found that a very small rotation of the chronometer every second will quickly cause the chronometer to become synchronized to that rotation because the escapement and balance system in the chronometer become resonant with the applied mechanical rotation. It is this resonance that affords the chronometer the ability to maintain “perfect” time as long as the atomic or GNSS signals are present.
If those signals are no longer present, such as if all power is lost for an extended period, the chronometer will revert to its natural rate. During normal operation, once per day at midnight UTC, the servo synchronization is turned off so that the natural rate of the chronometer can be measured versus the atomic or GNSS standard, and that error is printed on a paper tape. Should the electronic clock systems in the Time Traveler’s Clock be lost, the user can use these historical measurements to calculate the best time for navigation by applying the daily error to the time read on the chronometer, as was done in the past before satellite and atomic standards.
GPS clock advantages and deficiencies
The various global navigational satellite systems (GNSS systems, including the U.S. GPS, Russia’s Glonass, Europe’s Galileo, and China’s Beidou) rely on very high-precision timekeeping allowing a receiver to calculate its own position. Each satellite broadcasts its current time and its ephemeris (the parameters of its orbit). The receiver then compares the times received from multiple satellites, correct for relativistic timing frame differences, and perform a complex calculation to “multilaterate” its own position on earth.
In the process, the receiver also calculates the current time to a very high degree of accuracy, on the order of nano- or micro-seconds. A timing-rated receiver can provide true time accurate to within a few tens of nanoseconds.
When provided with an external frequency reference, the GNSS receiver can maintain the time (“holdover”) even if satellite signals are lost. By itself, the uBlox M8F receiver has a holdover precision of 1 x 10-7, or 8 milliseconds per day. If an atomic reference signal is supplied, then the holdover precision will be equal to the precision of the atomic reference signal (in the TTC, 1 x 10-10, equivalent to a few milliseconds per year).
Since these satellite signals are available almost anywhere on earth, the time provided by a GNSS receiver is the time used for most scientific, civil, and navigational purposes. Why would a user need to use precise time for navigation, given that he has a GNSS receiver giving precise latitude, longitude, and altitude data? The answer is that GNSS systems can be jammed or spoofed, resulting in inaccurate location calculations.
By keeping a reliable clock continually set to known good GNSS time, a navigator may use a sextant and navigational number tables to calculate his or her position, even if the GNSS signals are incorrect or unavailable. The Time Traveler’s Clock can be used for navigation because it has multiple time references, including atomic, quartz, and silicon-based clocks in addition to its mechanical chronometer.
When GNSS time signals are available, the quartz, silicon, and mechanical clocks are all kept synchronized to satellite time.
Silicon clock advantages and deficiencies
One of the redundant clocks contained in the TTC is an integrated-circuit chip (Dallas DS3231) that contains a temperature-corrected quartz oscillator of its own and an extremely low-power microprocessor that maintains correct time, even if its main power supply is lost, using a small backup battery. The lifetime of the backup battery is at least ten years, and it is only used when the normal power supply is not available.
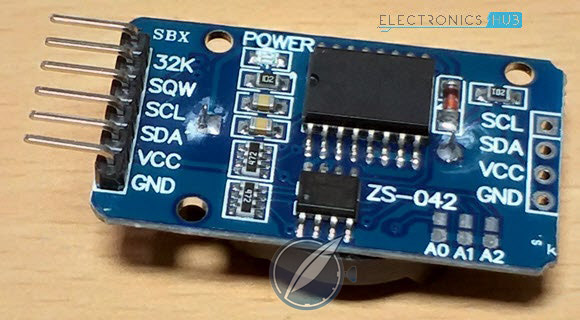
Real-time clock with DS3231 silicon integrated circuit
This integrated-circuit clock is not as accurate as an atomic or GPS clock, but it can serve as a timing reference if all else is lost, and it is a redundant clock to supplement the other quartz, atomic, and mechanical timekeeping systems. The integrated-circuit clock in the Dallas DS3231 chip is specified to be accurate to within one minute per year; DS3231 chips used in the Time Traveler’s Clock are individually tested and calibrated to achieve significantly improved timekeeping, on the order of seconds per year.
Atomic reference advantages and weaknesses
Atomic clocks use frequency standards based upon the oscillations of cesium or rubidium atoms. These are the most precise reference standards available that can be used in portable equipment. The “Miniature Atomic Clock” (MAC) and “Chip-Scale Atomic Clock” (CSAC) technology was developed by Jackson Laboratories. The current state-of-the-art devices are cesium CSAC modules such as the one used in the latest MCB version of the Time Traveler’s Clock.
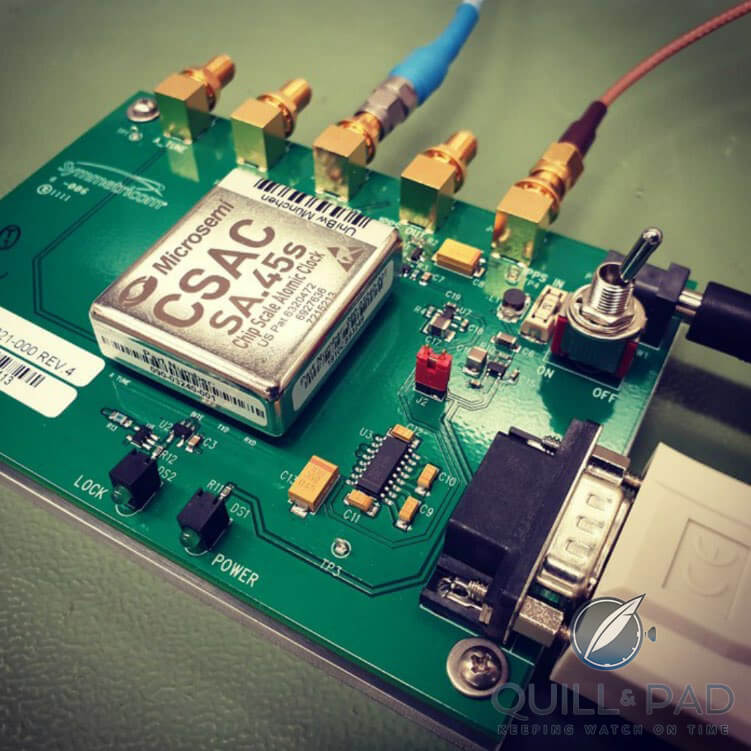
Cesium chip-scale atomic clock circuit board
The signal from this module, a 10 MHz reference, is supplied to the GNSS timing receiver so that if satellite signals are not available, the receiver will go into “holdover” mode with the precision of an atomic clock. The cesium CSAC modules have an accuracy of 1 x 10-10, which is equivalent to a few milliseconds per year. In the Time Traveler’s Clock this signal serves as the reference to not only the GNSS receiver, but also to the mechanical chronometer by way of the servo synchronization system.
Atomic clocks require continuous electrical current to maintain their accuracy. However, if power is restored after a temporary loss, the atomic clock module will re-establish its operation according to the last stored tuning data and once again begin to supply a stable reference signal to the GNSS receiver. During the time that the atomic reference is lost, the GNSS receiver will revert to its own internal quartz reference, which is precise to 1 x 10-7, or 8 milliseconds per day.
Time Traveler’s Clock functional modules
GPS receiver and clock
The most precise and accurate time signal currently available on earth is from the global constellations of navigational satellites, such as GPS (U.S.), Glonass (Russia), Beidou (China), and Galileo (European Union). To provide position data to GPS receivers, the satellites contain cesium atomic clocks that are continuously monitored and adjusted by their respective countries.
A GPS timing receiver can provide time to within as little as 10 nanoseconds as long as it has at least four satellites in view. When satellite reception is lost, the GPS timing receiver goes into “holdover” mode, in which it uses an internal quartz oscillator to maintain time. In holdover mode a GPS timing receiver by itself can maintain time to within 100 parts per billion, or a few seconds per year.
Cesium reference
The cesium reference oscillator is known as a “chip-scale atomic clock” (CSAC). In the CSAC, the oscillations of an isotope of cesium provide a “Stratum 1” 10 megahertz reference signal. This signal stabilizes the GPS receiver so that the clock will operate at the highest possible precision when a GPS signal is not present. The cesium CSAC is accurate to 1×10-11, which translates to less than a millisecond per year.
Silicon receiver and clock
An independent electronic clock is incorporated that utilizes a silicon real-time clock (RTC) chip to provide time in case the GPS signal is unavailable and the CSAC atomic clock fails or runs out of power. The RTC chip contains a temperature-compensated quartz oscillator accurate to within a few minutes per year. The RTC chip will operate independently from all the other systems by means of a lithium cell for several years. Its time and date are displayed on the display panel, which must be powered on, at least temporarily, to read the time.
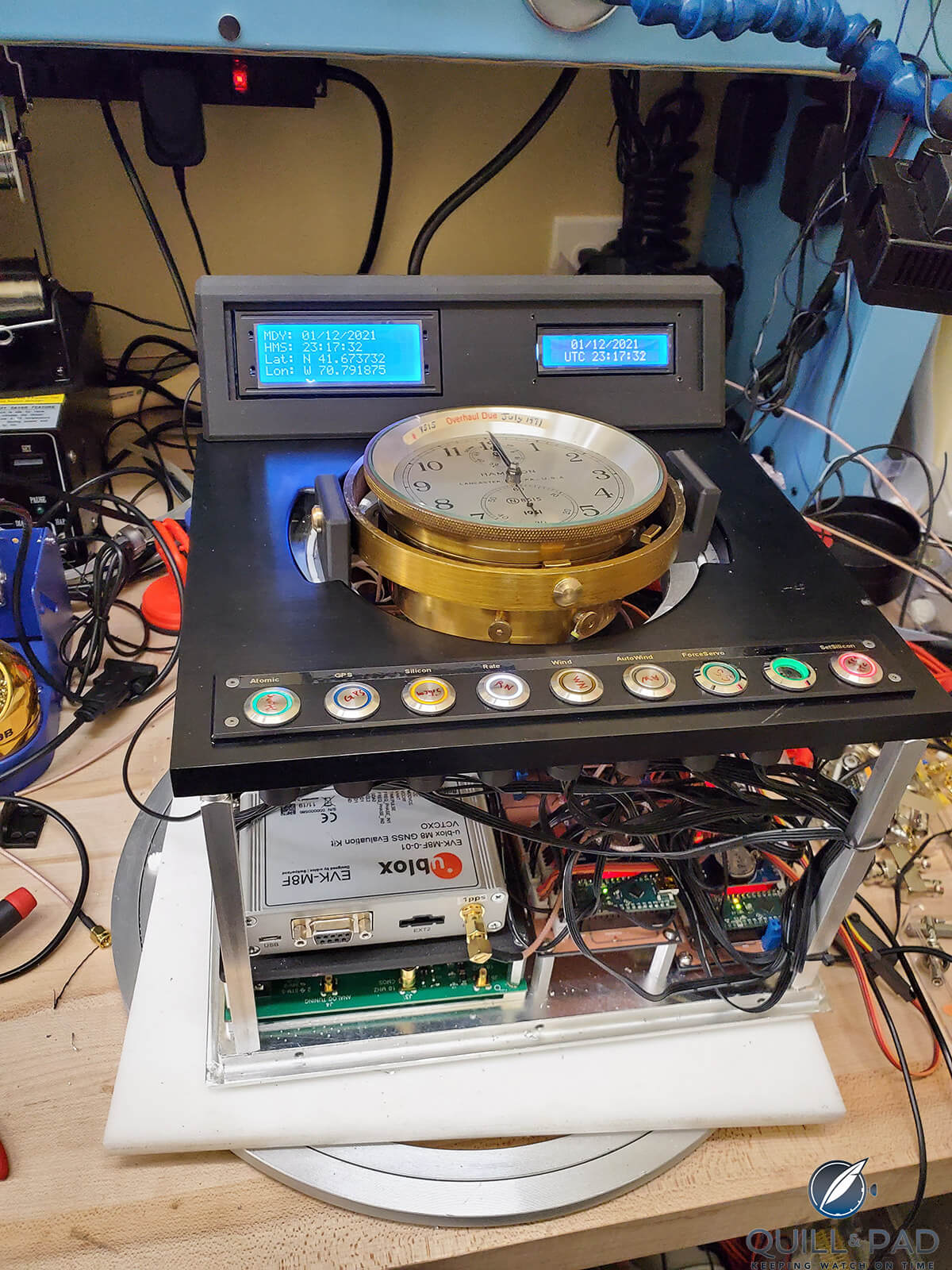
Time Traveler’s Clock uncased
Hamilton 21 Marine Chronometer
Before the advent of radio time signals and portable quartz clocks, ships at sea utilized a highly precise mechanical clock known as a chronometer to ascertain the ship’s longitude. Chronometers sufficiently accurate to provide navigational time at sea were first developed in the late eighteenth century by John Harrison. Over many years the design of marine chronometers advanced, culminating in the Hamilton Model 21 Marine Chronometer, developed during World War II by the Hamilton Watch Company. The Hamilton chronometer was an improvement over chronometers manufactured in Switzerland.
The U.S. Navy and U.S. Army used these chronometers until 1985, when they were replaced by quartz instruments. The Hamilton 21 has a typical rate stability of one part per million, equivalent to less than a minute per year. Generally marine chronometers are provided to the user with data on when it was set to correct time and how much the time can be expected to vary per day (rate error). For navigational use, the user must calculate the actual current time using the rate error and the number of days since the chronometer was set to correct time.
The Time Traveler’s Clock contains a servo mechanism that provides a mechanical twisting motion to the chronometer once per second to keep the chronometer synchronized with the GPS timing receiver (which in turn is stabilized by the cesium CSAC). Thus, the GPS clock, the cesium frequency reference, and the Hamilton chronometer are kept in resonance, and the chronometer will remain as accurate as the GPS and atomic references.
The Hamilton 21 chronometer in the Time Traveler’s Clock is equipped with an electronic winding system that winds the chronometer each hour to a consistent state of winding.
So that precise time could be calculated from the Hamilton chronometer even if the GPS and atomic references are not functioning (for example, if electrical power has been lost for longer than the backup batteries will maintain operation), the natural rate of the chronometer is measured once per day for a period of 30 minutes (at midnight), and the deviation of the mechanical clock from the GPS and atomic references is measured and printed out on the external printer. The user will thus have the best possible knowledge of the rate error of the chronometer, and the precise navigational time can be calculated from the number of days since it was last synchronized and the average rate error per day.
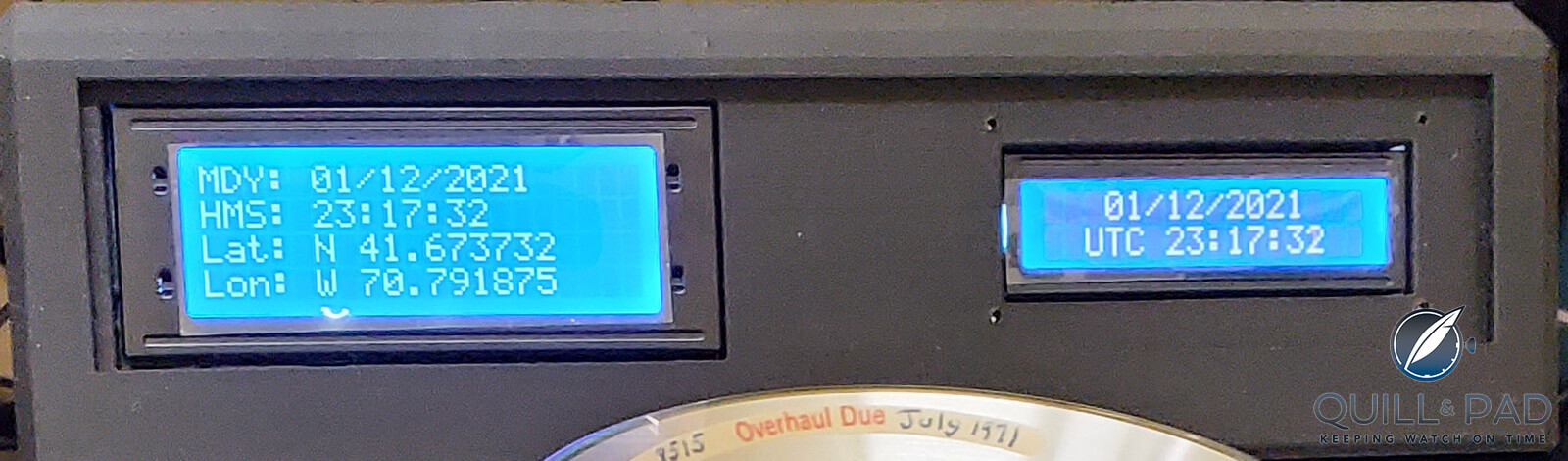
LCD display of the Time Traveler’s Clock
GPS and silicon clock display panel
The Time Traveler’s Clock has an electronic display panel that indicates the UTC time, date, latitude, and longitude from the GPS clock as well as UTC time and date as maintained by the silicon RTC clock.
UTC navigational time
There are many standards for time and date. Besides the standard local time zones, there is a basis time known as Coordinated Universal Time (UTC). UTC is defined as the time at the zero meridian that passes through Greenwich, UK. It is regulated by an international committee, the International Time Bureau. Periodically, leap seconds are added (or subtracted) from UTC to keep it more or less synchronous with earth’s rotation relative to the stars.
Since UTC is always within one half second of the earth’s rotation, it is the preferred time standard to be used for navigation. For that reason, the Time Traveler’s Clock uses UTC in all its displays and on the Hamilton chronometer.
So to when would you want to go?
For more information, please download the full text (including user manual) from quillandpad.com/wp-content/uploads/2022/05/Time-Travelers-Clock-User-Manual-with-images-RevG.pdf.
Quick Facts Time Traveler’s Clock
Case: 28 cm cube
Weight: 27 kg
Movements: Hamilton marine chronometer, Cesium atomic clock, GPS receiver
Functions: hours, minutes, seconds
Limitation: Tom Bales made this clock for himself, but if anyone is interested in commissioning one for themselves he would consider making another
Price guide: $120,000
You may also enjoy:
Measuring The Time Between The Seconds: The Truth Behind High-Frequency Movements
Leave a Reply
Want to join the discussion?Feel free to contribute!
Superb technical article with loads of interesting facts.
The idea of syncing all the clocks is magnificent. Never thought it can be so “easily” done.
Beautiful idea from a passionate mind.
Thank you for sharing the entire process.
Cheers,
Andrei